Research Activities: The following sections will briefly highlight some of our research activities. Heading link
(i) Materials Synthesis: Our expertise in material synthesis includes: (i) the chemical vapor transport (CVT) technique for the growth of pure and alloyed 3D crystals, (ii) mechanical exfoliation coupled to dry transfer technique to produce stacks of 2D materials with tailored properties, (iii) the chemical vapor deposition (CVD) technique to grow 2D materials with controlled grain boundaries, defects and interfaces, and (iv) solution-based processing techniques such as liquid-phase exfoliation and colloidal chemical synthesis to produce scalable dispersion of nanomaterials and quantum dots.
So far, we have synthesized single and multi-layer graphene, phosphorene, MXene, and a wide range of single phase and alloyed transition metal dichalcogenides (TMDCs) for various energy applications.
(ii) Heading link
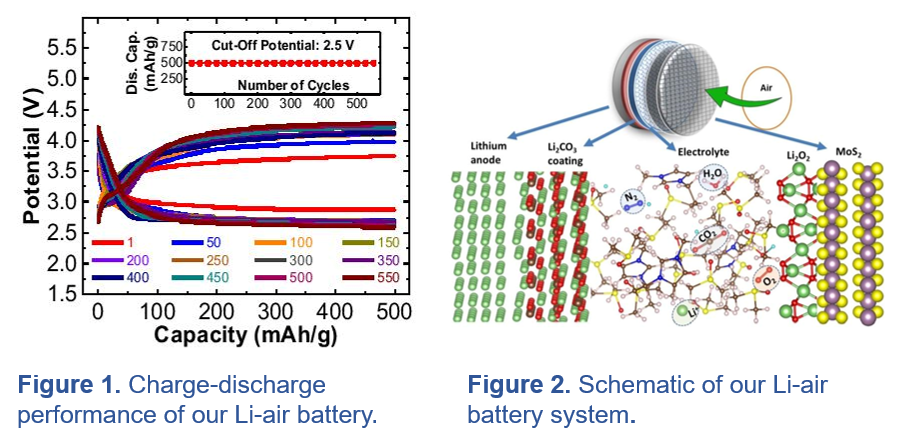
(ii) Energy Storage Systems for Electric Vehicle, Electric Flight, Wearable Robots and Bio-Medical Applications:
(A) Metal-Air Batteries that operate with real air (with all impurities) and can produce energy densities comparable to that of internal combustion engines. Metal-air batteries are considered an advanced solution for future energy requirements due to their remarkably high-energy density. For instance, the theoretical energy density of lithium-air batteries (3500 Wh/kg) is about one order of magnitude higher than that of commonly used lithium-ion batteries (~400 Wh/kg). In terms of cruising-speed range on passenger cars, the performance of lithium-air batteries is comparable to that of internal combustion engines. Thus far, the research on the lithium-air battery has been limited to the short life-cycle of lithium oxygen (Li-O2) batteries. The major problems with the existing Li-O2 systems is degradation of the anode, clogging of the cathode and instability of electrolyte. In the presence of air components, such as nitrogen (N2), carbon dioxide (CO2), and moisture (H2O), these issues become even more complex since a small concentration of such reagents could negatively influence reaction pathways and discharge-charge processes at the cathode. At UIC, we have developed the first generation of an operating lithium-air battery comprised of a lithium carbonate-based protected anode, a 2D molybdenum disulfide (MoS2) cathode, and ionic liquid/dimethyl sulfoxide electrolyte that work together with a long life of up to 700 cycles (published in Nature 2018). In order to implement this new concept beyond lithium-ion batteries, higher efficiency (lower charge overpotential), increased capacity, and longer cycle life are required.
Metal-CO2 Batteries: Heading link
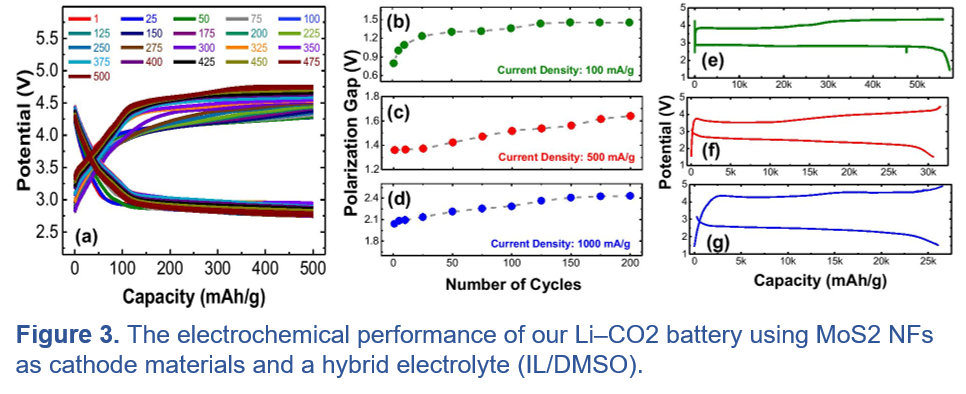
(B) Metal-CO2 Batteries: Although far less studied, lithium-CO2 batteries are attractive energy storage systems for fulfilling the demand for the future large-scale applications such as electric vehicles and grid systems due to their higher specific energy density (~1876 Wh/kg) compared to those of commonly used lithium-ion (~265 Wh/kg) and lead-acid (~30-40 Wh/kg) batteries. However, the major challenges with these batteries are the low cyclability and poor reversibility of discharge products (e.g., Li2CO3 and carbon) during the battery cycling. An ideal system must operate in carbon neutral conditions in order to reversibly balance the electrochemical reactions during discharge and charge processes. Recently, for the first time, we developed a carbon neutral and fully rechargeable Li-CO2 battery using carbon-free molybdenum disulfide nanoflakes as a cathode catalyst combined with a hybrid electrolyte based on ionic liquid and dimethyl sulfoxide. Our electrochemical measurements show remarkable reversible deep discharge/charge capacity of 60,000 mAh/g and superior long cycle life of 500, which is by far the highest capacity and best cycling stability reported in Li-CO2 batteries, respectively. The long cycle life demonstrates for the first time that C-O bond making and breaking chemical transformations can be used in energy storage systems, in addition to the widely studied alkali metal (Li-Na, K) -oxygen bond making and breaking transformations. While these proof of concept results show excellent cyclability of the Li-CO2 chemistry, the round-trip efficiency of the battery is still low (~60%). In order to implement this technology in grid systems and EVs, the stability and energy efficiency must be improved significantly.
(Photo)-Electrochemical Reactions to Produce Energy-Rich Chemicals: Heading link
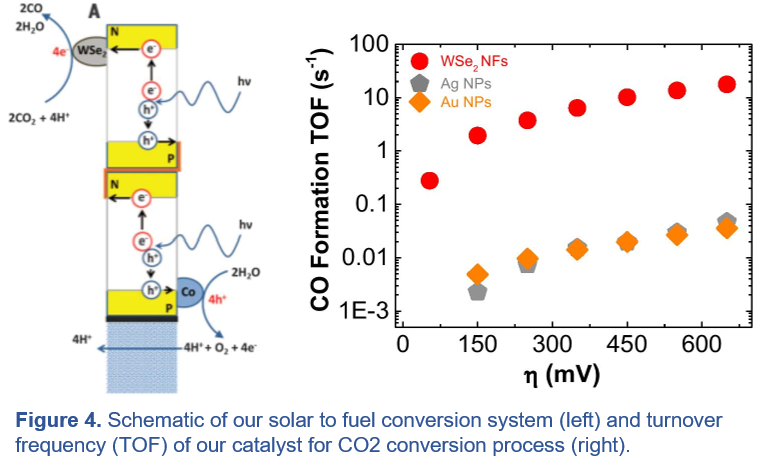
(iii) (Photo)-Electrochemical Reactions to Produce Energy-Rich Chemicals: Recently, we have discovered that the edge or surface states of transition metal dichalcogenides (TMDCs) in contact with ionic liquid electrolytes offers a new paradigm for electro-catalysis: materials with low work function, significant overlap of the d-band partial density of states with the Fermi energy, and an electrolyte “solvent” that protects rather than poisons the catalytic sites. In particular, we found that TMDCc/ionic liquid dual catalysts demonstrate three orders of magnitude higher catalytic activity for carbon dioxide (CO2) reduction reaction, far exceeding the performance of state-of-the-art catalysts such as oxide-derived materials, noble metal catalysts, and metal-carbon hybrid structures. We also developed the first generation of a solar-to-hydrocarbon energy storage system using a novel artificial leaf platform that mimics the photosynthesis process and converts CO2 to fuel using solar energy without any electricity input (published in Science 2016).
While we have identified unique features of the TMDC/IL liquid system for electrocatalysis, at present, little is known about the general electrochemical processes at the interface of an active edge atom of the TMDC catalyst with ionic liquid electrolyte, especially under realistic conditions and under an applied electric field. We perform both ex-situ and operando experiments to gain an in-depth understanding of electrochemical processes at the interface of solid/electrolyte interfaces. This information is used for the design of new materials.
Thermal Transport/Management in Micro/Nano-electronics: Heading link
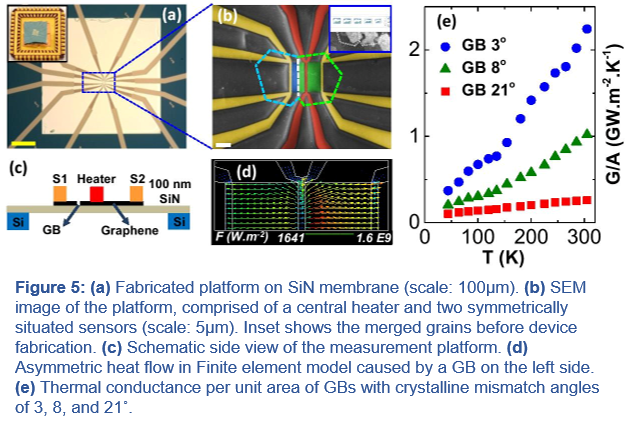
(iv) Thermal Transport/Management in Micro/Nano-electronics: Another effort in the lab is to obtain a deeper understanding of thermal transport in 2D materials for micro/nano- electronic applications. We study the limits set by the kinetics of dissipation and heat removal through various junctions and interfaces in 2D materials. In particular, we have developed novel platforms to measure thermal transport at micro/nano scales. This includes: (i) a unique electrical thermometry platform on a suspended silicon nitride (SiN) membrane (50-100nm thickness) that enables precise in-plane thermal transport measurements (Figure 5). With this platform, we have studied the thermal transport across an individual graphene grain boundary (GB) with different degree of mismatch angles (Figure 3e), (ii) a nanoscale thermometry platform using a combination of scanning transmission electron microscopy and electron energy-loss spectroscopy (STEM/EELS) to map the local temperature and thermal expansion coefficient in 2D materials using the temperature dependent Plasmon shifts. With this technique, we found an order of magnitude higher thermal expansion coefficient (TCE) for monolayer 2D TMDCs compared with their bulk limit, and (iii) a novel self-heating/self-sensing thermometry platform based on atomically-thin Ti3C2 MXene sheets that makes it possible, for the first time, to experimentally investigate the role of superstrate encapsulation on thermal transport at the interface of 2D materials with their 3D substrate.